The need to incorporate guidance on developing a public education and awareness programme as part of the Microbiological Water Quality Guidelines for Marine and Freshwater Recreational Areas was identified through feedback from councils and health authorities. Communicating risk, changing attitudes and behaviours towards monitoring programmes and water-quality issues, and inter-agency communication were among the main drivers for developing this appendix.
The goal of the Guidelines is to protect human health. In many cases, there is no ‘quick fix’ to bacteriological contamination of recreational waters, so one of the goals becomes risk communication. Relevant information must be communicated in an effective, efficient and timely way to the public, allowing them to make informed choices about whether to swim. Implementing a public education and awareness programme will help the public better understand what the guidelines mean to them, how they can minimise their chances of becoming sick as a result of swimming, and what – if anything – they can do to help improve their local water quality.
Designing and implementing your own programme
This appendix aims to provide councils and health agencies with a broad template for designing and implementing their own public education and awareness programme, to help achieve the broad aim of the guidelines:
to ensure that the public are informed of the microbiological health risks in time for them to make informed decisions about whether or not to enter the water.
Local variation and differences in the learning methods of the different stakeholder groups (e.g. informed versus uninformed) will to some extent determine the best means of delivering a message or forum for consultation. Taking the process in ‘bite-sized chunks’ and providing local context is a good basis for local programmes.
For additional information on designing, implementing and evaluating an environmental education programme, contact the education officer within your organisation or visit the Sustainable Management Fund website and download a copy of Environmental Education: A guide for programme providers – how to develop, implement and evaluate strategies and programmes.
Objectives
While the objective of the Guidelines may be met through risk communication in response to contamination events, mitigating contamination events requires a more detailed approach. Longer-term goals to clean up where poor water quality exists and where the community demands good water quality will inevitably require some action from individuals. In order to facilitate a change in attitudes and behaviours from the community, they need to be aware of their impact on water quality and what they can do to minimise those impacts.
Therefore, the two objectives of a public education and awareness programme are:
- to protect human health through the efficient, effective and timely communication of the health risks associated with using recreational waters
- to prevent, and where necessary isolate and remedy, bacteriological contamination of recreational waters.
Other objectives of this type of programme will be to:
- develop a base level of understanding of the issues within the community
- gain public support for the monitoring programme
- build confidence in the authority carrying out the monitoring
- accentuate the positive areas and use them as a selling point for the region
- build an image of responsibility for the council
- inform beach users of the immediate health risk
- manage community concern and public outrage
- maintain a responsible image
- encourage and facilitate community ownership and participation.
What is public education and awareness?
The following hierarchy defines public awareness and education:
- information: pamphlets, posters, etc.
- communication: public meetings, submissions, call centres, etc.
- education: consultation, developing knowledge, awareness, attitudes, values and skills that contribute to maintaining and improving the environment (Ministry for the Environment 1999).
The level at which councils and health agencies enter into a programme will depend on their statutory functions and their agreed role in implementing the guidelines. It will also depend on what level of participation or action the community expects. For example, one community may wish to be directly involved in a local monitoring programme, while others may be content to see weekly results published in the local paper.
Whatever the intention, this should be clear from the outset, and care taken not to raise expectations beyond this, as it will inevitably result in community backlash and mistrust in the council or health agency.
What is the environmental issue?
New Zealand has an extensive coastline and freshwater recreational area, which is used for a wide variety of recreational activities. Primarily usage is highest during the summer months (November to March), but there may be areas of the country or user groups that use recreational sites all year round. While water quality at New Zealand’s recreational beaches and freshwater sites is good most of the time, there are occasions when water may become contaminated with bacteria or viruses that cause respiratory or gastrointestinal illnesses, or skin infections. Susceptibility to illness is increased in those who have a compromised immune system, the very young and the elderly, and in severe cases may result in hospitalisation.
At the local/regional level specific environmental issues may vary. Consultation with local communities will help to identify these issues.
Who are the stakeholders?
It is important when designing an education strategy to identify the key stakeholders and how they use the beach in question. This will vary across the country and within each region or district, and may depend on the type of recreational site. There will also be variability over time within each user group; for example, there may be walkers who use the beach every day throughout the year, and those that only visit two or three times a season.
The main point is that baseline levels of awareness between and within stakeholder groups will vary considerably, as will the way in which they want/need to be involved in the programme. It is important to recognise this when designing a programme and to ensure the programme can be delivered at a variety of levels to suit the needs of those involved.
The following is a list of potential stakeholders, divided into those within monitoring agencies and those within the wider community.
Within council
Council staff
Council staff will inevitably have contact with the public, particularly after a pollution event when signs are posted advising against swimming. Frontline staff in particular should be trained appropriately to deal with possible irate members of the public, and be informed when they might expect such calls. Field staff may also come into contact with members of the public who are angry with the council for closing a beach. It is vital these staff are prepared for such encounters, and that the information they give the public is consistent with what other staff are communicating.
Councillors and potential councillors
It is important that councillors and potential councillors are aware of the issues surrounding beach water quality as they have a great deal of influence on what priority programmes are given. They are also directly affected by bad press and will need to be prepared should a pollution event result in media coverage.
Outside the council
Beach users
This group includes a variety of users that will vary locally. It is important to identify all user groups and how best to involve them in the programme, or at least how to communicate information. Some potential user groups are:
- local clubs (e.g. surf life-saving, Lions, waterski, sea scouts, walkers, kayakers)
- national and international groups (e.g. triathletes, boaties)
- schools
- parent networks
- iwi
- Pacific peoples’ groups
- other community groups
- ratepayers (including community boards).
These groups may be unaware of water-quality issues. They are also directly affected by poor water quality, beach closures and health impacts. The level of understanding and awareness should be established and programmes set accordingly. It is important that beach/river users are brought up to speed on the issue and kept informed throughout the monitoring season.
Local community (non-beach users)
Not everyone in the local community is interested in going to the beach. However, everyone will be affected by publicity about poor water quality at bathing beaches in their region. For example, homeowners may suffer a drop in property values and local businesses may notice a drop in trade. As with beach users it is important the local community is informed about the issue, and kept informed throughout the monitoring season.
Environmental interest groups
Environmental groups may have a greater level of understanding of the issues, so communicating with them at the same level as the above groups may be redundant or even detrimental. Instead, support from these groups should be sought. Environmental groups have the ability to sway the opinion of the general public, and if supportive of the council’s programme may be a real asset.
Media
The way the media interpret and report events will inevitably influence the community. Efforts should be made to ensure the message being reported by the media is accurate and factual, and that the positive aspects of the monitoring programme are highlighted.
Business
This includes:
- retail outlets, cafes, dairies, etc.
- tourism operators (including the Tourism Board)
- dive clubs.
Local businesses can be dramatically affected when a water-quality problem is identified. They can also be very influential in the community. Keeping them informed and getting their support for the monitoring programme at all stages is essential. Encouraging tourist operators to use clean beaches as a selling point will benefit the whole region, and provide an incentive to clean up beaches that experience water-quality problems.
Public health agencies, hospitals, GPs and laboratories
These groups need to be made aware that gastric and respiratory illnesses as well as skin infections can be caused through contact with bacteriologically contaminated bathing water. These agencies have a lot of contact with the public, so they are able to disseminate information effectively. This can be done through posters, fact sheets, and advice from staff.
What are the key outcomes sought?
If the objective of a public education and awareness programme is to encourage public participation, then the key outcomes of a programme should be developed in consultation with the community and be prepared to deliver what is jointly agreed. Developing outcomes in consultation with local communities will ensure they relate closely to regional or local issues.
On the other hand, if the intention is purely to communicate the results of your monitoring and to alert the public to specific contamination events, then consultation would be inappropriate, as it would raise false expectations and inevitably result in backlash.
Some key outcomes of a public education and awareness programme might be:
- increased understanding within the community of what the monitoring programme is about including:
- how, when, where and why
- monitoring is done
- what the indicator bacteria are and what their presence means
- costs and other resourcing issues
- how the results will be used
- the scale of the issue: put it into a local, national and international context
- sources of contamination and problem areas (e.g. stormwater, ageing infrastructure)
- causes of contamination are not always predictable
- even if a beach is ‘clean’, councils will monitor anyway to identify unexpected risks
- participation in ‘care’ groups
- a decrease in the number of swimming-related illnesses.
What methods should you use and what materials do you need?
The methods and materials used to get the message across will vary locally and with each stakeholder group. Consultation with the community will help to identify the level at which various groups wish to be involved and how they prefer to receive material and information. Consulting within the council will also help to identify existing programmes that can be tapped into to avoid reinventing the wheel and creating confusion.
The urgency of the message/information will also determine the methods used; e.g. if there is a contamination event and the message is a public health warning.
See Note H(xvii) for information on issuing health warnings.
The following are a variety of methods that can be used to deliver a range of materials:
- media – press (local and regional papers, magazines), radio
- a internet
- fact sheets (see Appendix 5)
- pamphlets
- community meetings
- school visits
- notices in local businesses
- notices/pamphlets in medical centres and hospital waiting rooms
- presentations to council committee meetings
- meetings with specific user groups
- establishing ‘care’ groups
- signs at beaches (see Appendix 3)
- 0800 numbers
- getting influential community members on board.
Practical considerations for delivering the programme
Identifying up front the practical aspects of how the programme will be delivered will avoid confusion and frustration when it comes time to implementing it. The following factors should be considered in advance of implementing the programme.
Who?
Clearly define who is responsible for delivering the programme. This is critical. Identify which agency will be responsible and the roles of individuals/officers within each organisation. The roles and responsibilities set out in Section B of Part I will help to identify which agencies and officers should be involved in a public education and awareness programme.
Clearly defined lines of communication within monitoring agencies, between agencies (regional council, territorial local authority and Medical Officer of Health) and to the public should be documented in the monitoring plan. This will include identifying the agency and officer responsible for notifying the public of health risks.
When?
Determining the timing for the programme is also very important, especially when communicating information about contamination events, which requires an immediate response. Starting the programme with sufficient time to lead in to the bathing season may also be useful. Timing of programmes will vary considerably across the country and will need to be decided locally.
As there is likely to be a number of agencies involved in the programme, it is important to define exactly when each of those agencies becomes involved and how.
Where?
Defining the extent of the programme will also affect how it is delivered and will depend on which agency is implementing the programme. Local authorities that co-ordinate programmes regionally may also wish to co-ordinate the public education and awareness programme. More specifically, this will affect what venues are used for public meetings, etc.
How will you monitor implementation and effectiveness?
It is important before beginning a programme such as this to establish a base level of awareness. This will enable progress to measured using this pre-programme information. User-group surveys, household surveys and council staff surveys are some of the methods that can be used to achieve this.
It is also important to review the programme on a regular basis. It will take time before changes in attitudes and behaviours are noticed, so a long-term plan for review is essential. Again, annual surveys are useful for this purpose.
Summary
An open, honest and transparent policy with the public is essential if the council is to gain support for their monitoring and remediation programmes. Authorities who have adopted this approach have received additional funding for infrastructure upgrades and increased community and media backing.
A number of councils have implemented programmes that utilise the community resource, such as training community volunteers to monitor water quality. Volunteers are trained and perform some monitoring functions. This approach has proven to be successful in raising levels of awareness and understanding of the issues surrounding beach water quality and in gaining support for monitoring programmes.
For further information on designing and implementing environmental education programmes, refer to the Ministry for the Environment’s Environmental Education: A guide for programme providers – how to develop, implement and evaluate environmental education programmes (1999).
In this appendix:
- section 1 summarises the two basic approaches that have been taken internationally and in New Zealand to the relation between recreational water users’ health risks and the degree of faecal contamination of the water, either by human or animal waste residues
- section 2 explains how the results of these studies have been incorporated into these Guidelines
- section 3 discusses some implementation issues.
Two basic approaches
Microbiological water-quality guidelines for recreational areas may be developed from two main strands of enquiry into health effects: epidemiological studies or quantitative risk assessment. In the former the focus is on direct measurement of health effects while in the latter the focus is first on pathogen concentrations, with health effects then being inferred using known dose-response relationships. Both types of study have been used to develop these guidelines: epidemiological for the marine waters and quantitative risk assessment for the freshwaters.
The main reason for choosing to base marine water guidelines on the epidemiological approach is that there is a wealth of results from such studies (Prüss 1998), including some for New Zealand (McBride, Salmond et al 1998). On the other hand very few such studies are available for freshwaters, and those that are available are nearly all confined to lakes, not rivers. However, we are now in possession of a large amount of data for pathogens and indicators in freshwaters and this has facilitated a quantitative risk assessment approach. Accordingly, the guidelines for freshwaters are based on this approach.
It is important to note that the wealth of results from international studies now available point overwhelmingly to an association between illness risk to recreational water users and the concentration of suitable faecal indicators (as reviewed by Prüss 1998). They show that careful studies are needed to reveal the relationship, particularly because many of the illnesses concerned are mild and no records are kept of their occurrence (i.e. they are not ‘notifiable’). [More severe illnesses (e.g. typhoid) do occur among swimmers at grossly polluted beaches (e.g. in Egypt, El-Sharkawi & Hassan 1979; Cabelli 1983a).] Furthermore, these illnesses include both gastrointestinal and respiratory categories (when sought, respiratory illness effects have often been found; e.g. Fattal et al 1986; Corbett et al 1993; Fleisher, Kay, Salmon et al 1996; McBride, Salmond et al 1998). [Ear, nose, throat and skin symptoms are also found, often being attributed to bather-to-bather transmission, rather than to micro-organisms of faecal origin.] While some studies fail to detect an association (e.g. New Jersey Department of Health 1989), this appears to be caused either by a lack of sufficient statistical power or a lack of an ‘exposure gradient’; i.e. a sufficient range of contamination in the waters studied (as was the case in the New Jersey study).
Epidemiological studies
In these studies one aims to discover the illness record of a number of water users who used a recreational site on a particular day – a day on which water-quality samples were also taken. This calls for an intensive effort in interviewing beach users on the sampling day, and following them up some days later to obtain a record of health effects; i.e. a record of self-diagnosis is obtained (medical records are not available for examination because most swimming-associated illnesses are mild and not notifiable). Associations between health effects and the swimmers versus non-swimmers are sought, to get an estimate of any swimming-associated, pollution-related effects.
The group of people interviewed may be those who have decided of their own volition to attend the beach, without knowing that a study was in progress, in which case it is an uncontrolled prospective study. On the other hand people may be recruited into the study and taken to a particular beach where they may swim, in which case it is a controlled cohort study. Most epidemiological studies have been of the former kind, but more recent efforts have used the controlled approach.
Results from controlled cohort studies have recently been endorsed by the World Health Organization and are being incorporated into their international guidance (WHO 2003). Accordingly, these New Zealand guidelines are also based on that approach, marking a distinct change from previous editions of the New Zealand guidelines (1992, 1998, 1999).
Nevertheless, comprehending the history of the development of these studies may be helpful, as given in the following sections.
US studies
Concerns about health risks to bathers in contaminated water in America in the 1940s led to the US Public Health Service conducting a series of uncontrolled prospective follow-up epidemiological studies at river, lake and coastal sites from 1948 to 1950 (Stevenson 1953). This was a large study that reported two statistically significant associations between swimmers’ health risk and water quality, measured as concentration of total coliforms. These two findings were for beaches on Lake Michigan, at Chicago, and for the Ohio River at Dayton, Kentucky (none were found for the two marine sites, in New York City).
The study design consisted of three major elements:
- approaching people at the beach to see whether they would agree to being questioned a few days later about their health
- making water-quality measurements at the beach on the same day as beach-goers are approached
- within a few days, questioning those who agreed to the follow-up as to any subsequent illness, as well as a host of other possibly confounding factors (such as other swimming, foods eaten, animal contact and household sickness).
Elements of the design of studies used to develop guidelines have been questioned over the years (e.g. Cabelli et al 1975; Moore 1975). This has included the following objections:
- ‘swimming’ did not necessarily include head immersion
- total coliforms are not very effective indexes of faecal pollution
- non-swimmers were not at the beach.
Such flaws were addressed in major further studies carried out in the US in the late 1970s and early 1980s for both marine waters (Cabelli 1983a) and freshwaters (Dufour 1984), with the motivation of providing public policy agencies with a relationship between “swimming-associated, pollution-related illness risk” and typical concentrations of faecal indicators (Cabelli et al 1975). The idea was that those agencies could use an ‘acceptable’ health risk to derive limits for faecal indicator concentrations. These researchers never did advocate any acceptable particular values of these risks. Motivation for the other studies was similar. Some workers have actually recommended guidelines or standards (Grabow et al 1989; Wyer et al 1999).
Other marine water epidemiological studies have been carried out in the USA, at New Jersey beaches (New Jersey Department of Health 1989) and at Santa Monica Bay, near stormwater outfalls (Haile et al 1996). The latter study did discover health effects related to proximity to the outfalls.
UK studies
Meanwhile, in the UK a view had held sway since the late 1950s that swimmers’ health risk had no relationship to degree of faecal contamination, unless beaches were “aesthetically revolting” (MRC 1959) or “aesthetically very unsatisfactory” (PHLS 1959). That view was challenged increasingly over the years as being untenable (e.g. Kay & McDonald 1986a; 1986b): it was derived from a retrospective case control study for two severe and notifiable illnesses, and generalised to all illnesses (many of which are not notifiable, and so lack any substantial data). Accordingly, some prospective uncontrolled studies have also been carried out in the UK also (Pike 1994).
[This report reviews two sets of UK studies: controlled studies at four beaches (subsequently reported by Kay et al 1994 and by Fleisher, Kay, Salmon et al 1996), and uncontrolled studies at another eight beaches (partly reported by Balarajan et al 1989 and Alexander et al 1992). A full open-literature paper on the eight-beach study has not been sighted.]
Another UK group has proposed that such studies are better carried out using a recruited, controlled cohort approach (Jones et al 1991). This cohort is randomly split into swimming and non-swimming parts. All eat the same foods. The swimmers are directed where to swim and for how long (immersing the head three times). Many water-quality measurements are made at the assigned swimming points. The follow-up consists of both self-reporting and clinical examinations.
The findings of these careful studies (endorsed by Telford 1996) have reported that faecal streptococci were related to adverse gastrointestinal health effects (threshold 32 per 100 mL, Kay et al 1994) and to acute febrile respiratory illness (threshold 60 per 100 mL, Fleisher, Kay, Salmon et al 1996). (This definition of respiratory illness requires an accompanying fever, and so is more stringent than that used in other studies, such as the one carried out in New Zealand by McBride, Salmond et al 1998.)
Note that the analysis of these UK studies postulates the existence of a threshold effect (a value of water quality below which there is no illness risk to swimmers whatsoever and above which there is). Analyses of other studies (e.g. Cabelli 1983a; Dufour 1984) have used continuous relationships between water quality and swimmers’ health. This is more consistent with the mixture of ages and health status of usual beach-goers: while an individual may have a particular threshold, it is unlikely that a whole population would share the same value. For that reason the UK analysis notes that the threshold should not be considered as an “absolute” value, noting that it may be set to a lower value were the study to have included a larger number of people (Wyer et al 1999).
Other relevant UK marine water studies are Brown et al 1987; Balarajan et al 1989 (see also its discussion by Hall & Rodrigues 1992); Alexander et al 1992 and Fewtrell et al 1994. Freshwater studies for canoeists and rafters have been reported by Fewtrell et al (1992, 1994) and by Lee et al (1997).
New Zealand studies
Prospective epidemiological studies were carried out at seven New Zealand beaches in the 1995/96 bathing season (McBride, Salmond et al 1998). This was particularly driven by concerns in the Auckland region about possible health effects at marine beaches.
Prior to statistical analysis each beach was placed into one of three categories: (i) impacted by human wastes, (ii) impacted by animal wastes, or (iii) pristine.
An association between enterococci concentration and respiratory illness symptoms among those entering the water was identified. [Some statistically significant associations with faecal coliform and E. coli concentrations were also noted. However their strength was lower because they tended not to rise through the quartiles of that indicator’s concentrations (whereas enterococci did so rise) and their relative risks were also lower than for enterococci.] This included “paddlers”, who entered the water but did not immerse their heads (e.g. tending small children). Relative risks in the highest enterococci quartile were rather high: 4.5 for the paddlers and 3.3 for long-duration swimmers. The unexpectedly limited range of beach contamination during the survey precluded the possibility of developing a detailed statistical model of health risk versus indicator density, as had been hoped. [The enterococci quartiles cut-offs were 1.5, 3.75 and 13 enterococci per 100 mL.] No substantial differences in illness risks were found between the two types of impacted beach, but the health risks for both were separable from that at pristine beaches.
Studies in other countries
Other relevant epidemiological studies have been conducted in the following countries (an asterisk * denotes a retrospective study):
- Australia: Corbett et al 1993; Harrington et al 1993
- Canada: EHD 1980; Seyfried et al 1985a and 1985b; Lightfoot 1989
- Egypt: Cabelli 1983a; El-Sharkawi & Hassan 1979*
- France: Foulon et al 1983; Ferley et al 1989*
- Holland: Medema et al 1995, 1997
- Hong Kong: Holmes 1989; Cheung et al 1990; Kueh et al 1995
- Israel: Fattal et al 1986, 1987, 1991
- New Zealand: Bandaranayake et al 1993; McBride, Salmond et al 1998
- Spain: Mujeriego et al 1982*; Mariño et al 1995b
- South Africa: Von Schirnding et al 1992; 1993
Comparing controlled and uncontrolled studies
Most of the studies listed above are of the uncontrolled type, so it is appropriate to consider which of the two main approaches to epidemiological studies is the better.
The first consideration is to note that there is no optimal way of conducting epidemiological surveys – each approach has its drawbacks (Lacey and Pike 1989). Clearly some are better than others, and flaws in older studies have been identified and remedied in later studies, such that a reasonably consistent body of evidence has now been gathered (Prüss 1998). In spite of the uncertainties involved in epidemiological studies with low attack rates, it is often still considered to be the best line of approach (cf. a risk calculation approach), where feasible (Ware 1990). This view is in spite of the fact that funds that would be spent on extensive interviewing in the epidemiological approach could be spent on a wider range of indicators and pathogens in a risk-calculation approach.
The controlled cohort prospective approach offers the most accurate methodology, minimising bias and providing for a balanced matching of swimmers and non-swimmers (Fleisher 1990b; Fleisher, Jones, Kay & Morano 1993). However, its use does require pre-publicity, which may cause enhanced self-reporting rates (Wheeler & Alexander 1992). Also, the cohort used (healthy adult volunteers) is not typical of the usual beach-going population (which includes many ages and variable health status), and the type of swimming activity may not be typical either – especially for high wave-energy New Zealand beaches where boogey boarding and body surfing are so popular. (This is important, because close-to-shore waters are often the more polluted.)
The uncontrolled cohort prospective approach offers the advantages of minimal pre-publicity and of using the actual population using the beach. It suffers from difficulties in assigning water quality to particular swimmers (according to where they swam) and in having a somewhat unbalanced set of swimmers and non-swimmers.
The view taken in these guidelines is that, given the endorsement of the WHO (2003), the results of the carefully conducted controlled cohort UK studies will be used as a basis for marine beach grading. Note that the illness rates reported by these studies, both for swimmers and for non-swimmers, tend to be higher than for uncontrolled studies.
The quantitative risk assessment approach
Some early work leading to the setting of water-quality microbiological standards and guidelines was based on a risk calculation approach. For example, Streeter (1951) calculated an individual’s risk of contracting typhoid fever or “diarrhoea-enteritis” assuming a concentration of 1000 total coliforms per 100 mL. For 90 consecutive daily exposures to this concentration the calculated risks were 1:950 and 1:50 for the two illnesses, respectively. Also, Furfari (1968) reports how shellfish standards have been calculated. [By requiring that no more than 50% of 1 mL samples were positive for coliforms, that being equivalent to an MPN of about 70 per 100 mL.]
Recent developments in this approach have noted a number of shortcomings (see especially Haas et al 1999). In particular:
- water users experience a range of concentrations of pathogens and indicators from one day to the next, and even within a day
- they also have variable rates of ingestion or inhalation of water, and for varying times
- dose-response relationships (between illness risk and indicator density) have been lacking.
With the advent of powerful computer technology these issues can now be addressed relatively easily, using ‘Monte Carlo’ mathematical modelling. This is known as the Quantitative Risk Assessment (QRA) approach. Historical data is used to assign statistical distributions to the ingestion/inhalation rates, duration of exposure, and the concentration of pathogens in the water. Then a random sample is taken from each distribution to calculate the dose, which is then turned into infection or illness probabilities, or into cases, using a dose-response curve. This sampling is done many times over to simulate a large population being exposed to beach water that may, on some occasions, be contaminated.
When this is done for a population of people at a given beach (not dispersed over many beaches) the end result is that on a majority of occasions there are no cases of infection, but on a few occasions (when the contamination is unusually high and recreational water contact actually occurs) a number of infections, and hence illness, could occur.
The greatest weakness of this approach is the paucity of dose?response information. However, a surprising amount is now available – as reviewed by Teunis et al (1996) for gastrointestinal pathogens; McBride et al (2002) also include a review of material for adenovirus respiratory pathogens, only some of which is covered by Haas et al (1999).
The New Zealand study
The QRA approach has been reported in some detail for New Zealand freshwater recreational waters, as a consequence of a large national study at 22 river and three lake sites, in which five indicators and six pathogens were sampled fortnightly for 15 months in the period 1998–2000 (McBride et al 2002). The main reason for adopting the QRA approach was that epidemiological studies – either controlled or uncontrolled – were held to be unfeasible. While McBride et al (1996) concluded that a controlled cohort study would in fact be feasible, two subsequent considerations ruled it out. First was the difficulty in recruiting suitable cohorts within proper ethical requirements, and second was the paucity of available data on pathogens and indicators in New Zealand freshwaters. Given that there were many indications that freshwaters were rather more contaminated than marine beaches, it seemed prudent to attempt to plug this gap and to use the results in a QRA approach.
That study produced a wealth of information on the distribution of pathogens and indicators. In particular it found that Campylobacter was present in 60% of all samples, and that human enterovirus and/or human adenovirus were present in 54% of samples. Concentrations and occurrences of Salmonellae, Giardia cysts and Cryptosporidium oocysts were low. Catchments impacted predominantly by birds were the most contaminated, followed by those in which the dominant impact was from dairying or sheep farming. The degree of contamination was strongly related to the turbidity of the water.
In essence the study confirmed the continued use of E. coli as a faecal health-risk indicator, at least so far as Campylobacter is concerned. [Campylobacteriosis illness forms more than half of all reported notifiable illnesses in New Zealand, in recent times being around 300 cases per 100,000 population per annum (see the New Zealand Public Health Reports, published by ESR).] Unfortunately correlations between this indicator and the two virus groups examined (human enterovirus and human adenovirus) were very poor, as was the case for all other indicators examined – somatic coliphage, FRNA phage and C. perfingens spores. Also, correlation between the enterovirus and adenovirus groups was poor (enterovirus has also been used as a general virus indicator).
Accordingly, in the health-risk modelling particular attention was paid to Campylobacter infection as well as enterovirus and adenovirus infections. [The endpoint of this analsyis was taken as infection, not illness, principally because once infection rates are controlled to low levels, so too will be the illness rate. Also there are some severe practical difficulties in determining the probability of illness (given infection has occurred) as a function of dose (Teunis et al 1999).] Of the two virus groups, dose-response information suggests strongly that adenovirus is much the more infective, with risk profiles for a given beach being very similar to that for Campylobacter infections.
Deriving the guidelines
The following sections describe the basis of the guidelines for marine water and then for freshwater, both for the grading of beaches and for their ongoing surveillance. In each case a subsection describes the changes from previous guidelines.
General considerations
Since 1999 the WHO has favoured using 95 percentiles of microbiological concentrations for grading beaches via a Microbiological Assessment Category (first proposed in the Annapolis Protocol, WHO 1999). That approach is adopted here; i.e. these 2003 guidelines incorporate a risk-based approach to monitoring recreational waters, in addition to single samples as used in previous guidelines. The purpose of incorporating risk assessment is to overcome the constraints of these previous guidelines, as discussed in Section C.
Taking risk into consideration when assessing a site is achieved via a grading process, combining historical microbiological results and sanitary inspection information to give an overall Suitability for Recreation Grade. This grade provides an assessment of the condition of a site at any given time, while single samples are used to identify any immediate health risk.
The WHO provides no guidance for surveillance values; their derivation is explained below.
See Note G(x) for discussion on the Annapolis Protocol.
Marine waters
Beach grading
Results of the UK controlled epidemiological trials have been used to develop a four-category scale, as shown in Table H1. The essential results are those for gastrointestinal illness (as reported by Kay et al 1994; Wyer et al 1999), but also accompanied by the results for respiratory illness reported by Fleisher, Kay, Salmon et al (1996). In essence 95 percentile enterococci values have been identified relating to cut-off gastrointestinal risks of 1%, 5%, and 10%. The associated respiratory illness risk cut-offs are 0.3%, 1.9% and 3.9%. [Note that these are risks of acute febrile respiratory illness (AFRI). In the New Zealand study (McBride, Salmond et al 1998), and in other studies, a less strict definition of respiratory illness has been used – essentially not requiring fever as an accompanying symptom. This was a deliberate choice in the New Zealand study, given our incidence of asthma. Accordingly, the risks of more respiratory illness would be higher than these AFRI results indicate.] Readers are directed to these publications for details of the modelling approach used to derive these values.
Beach surveillance
Neither the WHO (2003) nor the authors of the UK studies on which the WHO guidance is based give any guidance for deriving surveillance values. Accordingly, we have used results from previous uncontrolled epidemiological studies, in particular those of Cabelli (1983a), also used in previous versions of the guidelines. While this could be argued to be somewhat dislocated, it has the advantage of maintaining good continuity with past practice.
These values are obtained by assuming that the distribution of enterococci is lognormal, the standard deviation of the logarithms of enterococci concentration is 0.7 (a reasonable average of available data) and the enterococci concentration is at the previous limit of a median of 35 per 100 mL (corresponding, under Cabelli’s model, to a swimming-associated risk of 19 per 1000 bathing events). Then the alert (amber) and action (red) limits are taken as the 80% and 90% upper one-sided tolerance limits for that distribution. [In the one-sided case, tolerance limits and confidence limits are operationally identical; this is not so in a two-sided case.] These figures may be simply calculated as 136 and 276 enterococci per 100 mL. [The 80 percentile and 90 percentile abscissae of the unit normal distribution are 0.8416 and 1.2816 respectively. So the one-sided 80% upper tolerance limit is 35x100.8416x0.7 = 135.9. Similarly the 90% upper tolerance limit is 35x101.2816x0.7 = 276.2.] Having regard to the uncertainty in estimating the standard deviation (of the logarithms) it seems appropriate to round these figures to 140 and 280 enterococci per 100 mL.
It is important to note that while this calculation is based on the assumption that waters are marginal for compliance with the median value, this does not mean that the alert and action limits will only be exceeded once the health risk rises above 19 per 1000 swimming events. In fact the alert and action limits begin to be exceeded when the true median concentration is someway below 19 per 1000 events. The unfortunate fact is that we can only associate particular risks with average or median values of enterococci; it is impossible to associate risks with any particular enterococci value. All that can be said is that if the alert and action levels are not exceeded then the illness risks are some way below 19 per 1000 recreational events.
Changes from previous guidelines
The previous guidelines were based on Cabelli’s uncontrolled study, as implemented by the USEPA. That is a median enterococci concentration of 35 per 100 mL, corresponding to a swimming-associated illness risk of 19 per 1000 swimmers. Also the alert and action limits have been rounded from 136 and 277 enterococci per 100 mL to 140 and 280 enterococci per 100 mL.
Background to the changes
It is of interest to note that the previous guidelines were not based on an explicit adoption of an ‘acceptable illness risk’ of 19 per 1000 bathers. What in fact happened is that the USEPA first proposed that criteria be based on a maximum acceptable illness risk of six per 1000 bathers (in the Federal Register 1984), corresponding to a geometric mean (or median) concentration of three enterococci per 100 mL. Submissions on the proposal noted this limit was so low as to be impractical (i.e. unattainable) in near-shore coastal environments. The counter-argument was made that the previous limit (geometric mean 200 faecal coliforms per 100 mL) had appeared to work satisfactorily, so why not use corresponding limits of enterococci? This argument was accepted by the USEPA, which used ratios of faecal coliforms versus enterococci to establish correspondences between faecal coliforms and the new preferred enterococci indicator (Favero 1985; USEPA 1986a), i.e. 200 faecal coliforms per 100 mL corresponded to 35 enterococci per 100 mL in coastal waters. Using Cabelli’s relationship, this corresponds to an illness risk of 19 per 1000 bathing events.
That is, the ‘acceptable’ illness risks were not chosen a priori [It would be very odd if they had been, since 19 is not a number that the public might generally adopt as being ‘acceptable’.] but were calculated, once it was decided that risks corresponding to the previous criteria (200 faecal coliforms per 100 mL, as a geometric mean) should be adopted.
In contrast, these new guidelines are based on an explicit choice of acceptable risks.
Freshwaters
Beach grading
For consistency with the marine grading system, the same basic structure is used for the Microbiological Assessment Category, i.e. a four-category scale. However, the risk cut-offs have been set at lower values: 0.1%, 1% and 5%. There are two reasons for adopting these lower values. First, they are based on Campylobacter infection only: we simply lack credible information to develop risk figures for other illnesses. However, as this particular infection is important in the New Zealand setting these risks have been taken as a suitably precautionary approach. Second, the upper level (5%) represents a doubling of the background infection rate and this has been viewed as a tolerable upper limit.
These risks have been calculated in the New Zealand QRA study (McBride, Till, Ryan et al 2002) for Campylobacter infection, results for which as given in Table H2.
The derivation of that table’s figures relied on the moderate correlations found in the New Zealand study between Campylobacter and E. coli concentrations. The values of calculated Campylobacter concentrations corresponding to the risk cut-offs were obtained. These corresponded to Campylobacter percentiles of 55%, 70% and 80–85%. [Using the results for all beaches and all times in Table A3.7.3 of McBride et al (2002).] The corresponding percentiles of E. coli were then read from its distribution, being 131, 261 and about 550 E. coli per 100 mL. These values were then rounded to the values in Table H2 (viz, 130, 260, 550 E. coli respectively).
Beach surveillance
In the absence of better information, the alert and action levels have been taken as the second and third E. coli cut-offs for beach grading.
Changes from previous guidelines
The previous guidelines were based on the Dufour (1984) study, as implemented by the USEPA. That is a median E. coli concentration of 126 per 100 mL, corresponding to a swimming-associated illness risk of 8 per 100 bathers. Also, the alert and action limits have been rounded from 273 and 410 E. coli per 100 mL to 260 and 550 E. coli per 100 mL.
Background to the changes
Once again, the previous guidelines were not based on an explicit adoption of an ‘acceptable illness risk’ of 8 per 100 bathers. The reasoning was entirely similar to that given above for marine waters, in which 200 faecal coliforms per 100 mL was found to be equivalent to 126 E. coli per 100 mL. That is, the ‘acceptable’ illness risks were not chosen a priori but were calculated, once it was decided that risks corresponding to the previous criteria (200 faecal coliforms per 100 mL, as a geometric mean) should be adopted.
In contrast, these new guidelines are based on an explicit choice of acceptable risks.
Implementation issues
Change in sampling depth
The provisional guidelines (Department of Health 1992) required sampling at adult chest depth, the reason being that this was the sampling depth used by the two studies on which the guidelines were based (Cabelli 1983a; Dufour 1984). This has now been changed to sampling at 0.5 m. This was partly driven by a concern expressed by some New Zealand sampling teams about the safety of sampling in New Zealand’s high-energy coastal waters. But it was also done in the light of other studies. Controlled-cohort studies in the UK have shown very clearly that swimming-associated illness risks are related to water quality measured at and only at the swimming location (Fleisher, Jones, Kay, Stanwell-Smith et al 1993; Kay et al 1994).
The 1998 guidelines advocated sampling at 0.5 m. This considered children, who use shallower-depth water and may be more susceptible to illness than adults, and that adults are also exposed to shallower water. The technical justification for the change in sampling depth is as follows.
In the New Zealand study, bathers were exposed to a variety of depths. Water samples were taken at both chest and knee depths. Using a median of 35/100 mL and taking into consideration both gastrointestinal and respiratory illnesses (not considered in studies on which the 1992 provisional guidelines were based), the maximum level of risk to swimmers at a depth of 0.5 m (previously applied to chest depth) remained at 19/1000. Therefore the risk level of 19/1000 relates to the number (35/100 mL) of indicator bacteria measured at any given depth.
However, concentrations of indicator bacteria at 0.5 m are nearly always found to be more than at chest depth (and considerably more than water beyond breaking waves). In fact the New Zealand study found that, on average, the values found at chest depth were about 50% lower than those found at 0.5 m.
Single category for bathing areas
The provisional guidelines followed the USEPA (1986b) in using four separate categories for maximum limits for indicator bacteria. These four categories correspond to four levels of beach usage (infrequent use, light use, moderate use and designated bathing beaches). This was based on the notion of minimising community risk rather than of minimising individual risk, a factor often criticised (Fleisher 1991). The adjustment of the limits to one category of use is based on the principle that the level of risk at a beach is independent of its popularity.
Difference between faecal streptococci and enterococci
The faecal streptococcus group consists of a number of species of the genus Streptococcus, such as S. faecalis, S. faecium, S. avium, S. bovis, S. equinus and S. gallinarum. They have all been isolated from the faeces of warm-blooded animals, and S. avium and S. gallinarum occur in poultry. S. bovis and S. equinus are residents of the bovine and equine intestinal tracts and, although detectable in their faeces, do not survive well outside the animal host and die off rapidly once exposed to aquatic environments. The faecal streptococci have been used in many of the European, UK and Australian studies of water pollution.
The enterococcus group is a subgroup of the faecal streptococci that includes S. faecalis, S. faecium, S. gallinarum and S. avium. Procedures for the isolation and identification of the enterococcus group from aquatic environments have been well validated as identifying this group as a valuable indicator for determining the extent of faecal contamination of recreational marine waters. Studies at marine and freshwater bathing beaches have indicated that swimming-associated gastroenteritis and respiratory illness can be related directly to the quality of the bathing water, and that enterococci are the most efficient bacterial indicator for marine water quality (Cabelli, Dufour, et al 1983; Dufour 1984; McBride, Salmond et al 1998). The enterococci have been used predominantly in American studies of water pollution, but also in Europe. Studies in New Zealand using enterococci as the indicator of choice form the basis of the current recreational water-quality guidelines for the marine environment.
Urban and rural run-off
Rural and urban run-off can contain both human and animal faeces. The catchment type will reflect the likely proportions of each. Animals can carry pathogens that may be passed on to humans (zoonoses) such as Giardia, Salmonella, Campylobacter, Cryptosporidium (Donnison and Ross 1999) and verotoxic E. coli (E. coli 0157). In times of high rainfall the pathogens present in animal faeces can be transferred into waterways via stormwater drains or overland flow.
The results of some studies (Calderon et al 1991, as interpreted by McBride 1993; McBride, Salmond et al 1998) indicate that illness risks posed by animal versus human faecal material should be considered to be equivalent, although the first Hong Kong study results are less clear (Cheung et al 1990).
For urban catchments, at least, indicators at beaches could be elevated by inflow (wrong connections) and infiltration of stormwater into the sewer system, leading to sewer overflows which then contaminate stormwater conduits (streams, channels, direct pipes, etc). These contamination events are probably dependent on rain intensity and may be able to be quantified. Also, animal faecal matter, soil and vegetative indicator inputs and other undefined sources can contaminate stormwater itself (without sewer overflow). Directly leaking sewers (termed ‘exfiltration’) can occur in any weather, contributing significantly to infiltration when it does rain or when groundwater levels rise.
Stormwater from unreticulated (but not necessarily ‘rural’) areas may contain faecal indicators from direct surface run-off containing animal or bird faecal matter and vegetative inputs, direct stock access to waterways, direct stormwater delivery to the coastal marine area, and indirectly via streams, all of which are believed to constitute a risk to human health.
Only one study (Haile et al 1996) has been conducted in waters impacted by direct urban run-off (storm drains). The rates of illnesses presented were similar to those of other studies conducted in waters contaminated with domestic sewage. However, Ferguson et al (1996) found that increased levels of faecal coliforms, faecal streptococci, Clostridium perfringens spores, Giardia and Cryptosporidium occurred in an urban estuary after rainfall. Gibson et al 1998 found that combined sewer overflows contributed increased Cryptosporidium and Giardia in both dry and (particularly) wet weather. Data from a study by Grohmann et al (1993) suggests that stormwater was a source of virus contamination in river and coastal water systems. It is therefore very likely that increased indicator levels identified at a beach following rainfall are indicating increased pathogen levels, and increased risk.
During the epidemiological study in New Zealand (see below), insufficient questionnaires were filled out immediately following rainfall events because people were not swimming at those times. However, the microbiological analyses were still conducted and the data shows that indicator levels go up at times of rainfall as a result of subsequent run-off.
The original claim from Cabelli was that rural point sources probably do not pose as great a risk as sources of human wastes. The advent of findings of Giardia, Cryptosporidium and Campylobacter, and the reinterpretation of the Connecticut rural swimming-pond study (McBride 1993), confounds this. Furthermore, the 1995 New Zealand marine beaches study (McBride, Salmond et al 1998) showed no statistically significant difference between beaches with urban versus rural impacts; indeed, the illness risks actually measured for those two impacts were very similar.
Illness: indicator relationships
Many more studies have been conducted for marine waters than for freshwaters. For marine waters the indicator of choice has usually been faecal streptococci (especially in the UK, Europe and Australia) and its subset, enterococci (especially in the US and New Zealand). For freshwaters, E. coli is usually the indicator of choice.
The essential idea behind the use of bacterial faecal indicators is that they may best represent overall pathogenicity of the water, as they may be well correlated to some pathogens but poorly correlated to others (e.g. protozoa and viruses). This lack of correlation has been shown in many studies (e.g. Elliott and Colwell 1985; Grabow et al 1989; Ashbolt et al 1993; Ferguson et al 1996). It must always be remembered that only a portion of the pathogens are ever measured, and some (e.g. Norwalk-like viruses) cannot be enumerated routinely.
Both the controlled and uncontrolled cohort approaches have identified significant (in the statistical and social senses of that word) increasing relationships between the risk of illness to swimmers in water containing faecal residues and the concentration of one or more bacterial faecal indicators. While many of the illness-causing organisms are not bacterial (e.g. viruses and protozoan cysts) and may not be well correlated to the bacterial indicator(s), the general form of this relationship is found among many studies (as reviewed recently by Prüss 1998).
Symptoms are reported to increase with increased exposure to water and to aerosols (spray from breaking waves). Studies have increasingly found significant relationships between respiratory illness risk and a bacterial indicator (Balarajan et al 1989; Cheung et al 1990; Fewtrell et al 1992; Corbett et al 1993; Fleisher, Kay, Salmon et al 1996; McBride, Salmond et al 1998), as well as gastrointestinal illness. Skin rash, and eye and ear complaints seem to be related more to the presence of other bathers (bather-to-bather transmission occurs) than to degree of faecal contamination.
Children may be more susceptible to illness than adults, although they do tend to swim in waters that are shallower and are hence more polluted.
Interpreting human health risk
Investigations on the possible relationship between water contamination and human health risk (e.g. of swimmers) have to account for various uncertainties. These arise because we only have a small number of samples from which to characterise the degree of contamination of the water the swimmers use, and we have health status information from only a tiny part of the whole population of swimmers. [As well as some non-swimmers, who are used as controls, it is the difference in illness risk between swimmers and non-swimmers that we are interested in.] If we took the measured relationship to be the true relationship, these uncertainties would be ignored and our relationship would have a high chance of being incorrect. Statistical methods allow us to account for these uncertainties.
It is traditional to use ‘null hypothesis tests’ to account for uncertainties. These test the hypothesis that there is no association between the indicator concentration and the swimmers’ risk of illness. [That is, one posits a ‘null’ hypothesis – that the correlation is exactly zero.] If the association (measured by a correlation coefficient or by a regression coefficient) indicated by the data is in some sense strong enough [The computed p-value would be less than half of the a priori significance level (usually denoted by a). The p-value is the probability of getting a correlation at least as extreme as has been obtained, if the null hypothesis were true.], one concludes that the null hypothesis should be rejected and that a ‘statistically significant’ result has been found. In this case, the true association is estimated by that found in the sample data. The level of uncertainty in this estimate is indicated by an interval (usually the 95% confidence interval) within which we might usually expect the underlying true value to lie, 95% of the time.
On the other hand, failing to reject the hypothesis simply means that the observed data is not inconsistent with the null hypothesis. It does not mean that we can regard the null hypothesis as being true, i.e. that there is no association at all. There may well be an association, but our sample data is held to be insufficient to reliably infer either this or its magnitude. This can be either because there is too much variability in the data, or that insufficient data was collected given its degree of variability. Increasing the size of the sample data set may identify an association – unless the variability continues.
A cautionary note: the form of words used by some authors to interpret a negative result for a null hypothesis test can be ambiguous (McBride, Loftis et al 1993). For example, in reporting on a study of bathers’ illness risks in a freshwater pond contaminated by animal faecal material (Calderon et al 1991), a null hypothesis test (only just) failed to reach ‘statistical significance’. This led the authors to state that there was “no association” between swimmers’ illness risk and animal faecal contamination.
This conclusion had quite dramatic consequences because it was thought to support the idea that bathers’ illness risks from exposure to animal faecal residues are much lower than for exposure to human faecal residues, and perhaps did not even exist (as is implied by the phrase “no association”). But if more swimmers had been included it is entirely plausible that the test result would have been statistically significant (McBride, Loftis, et al 1993). [Technically, the ‘power of the test’ increases with the number of data used, making it more likely that a null hypothesis will be rejected.]
In fact the measured relationship was quite large, but the data’s variability and limited size meant that the associated null hypothesis test failed to attain statistical significance. In other words, failure to attain statistical significance does not necessarily imply that the relationship tested lacks practical significance. The actual results found can be used, with results from other studies, in some kind of meta-analysis. [Meta-analysis refers to pooling data from a number of studies to reanalyse the relationship.]
Temporary health warning sign
These signs should be erected at entrances to sites as soon as possible following contamination events. Dimensions for these signs may vary, but they should be clearly visible from site access points.
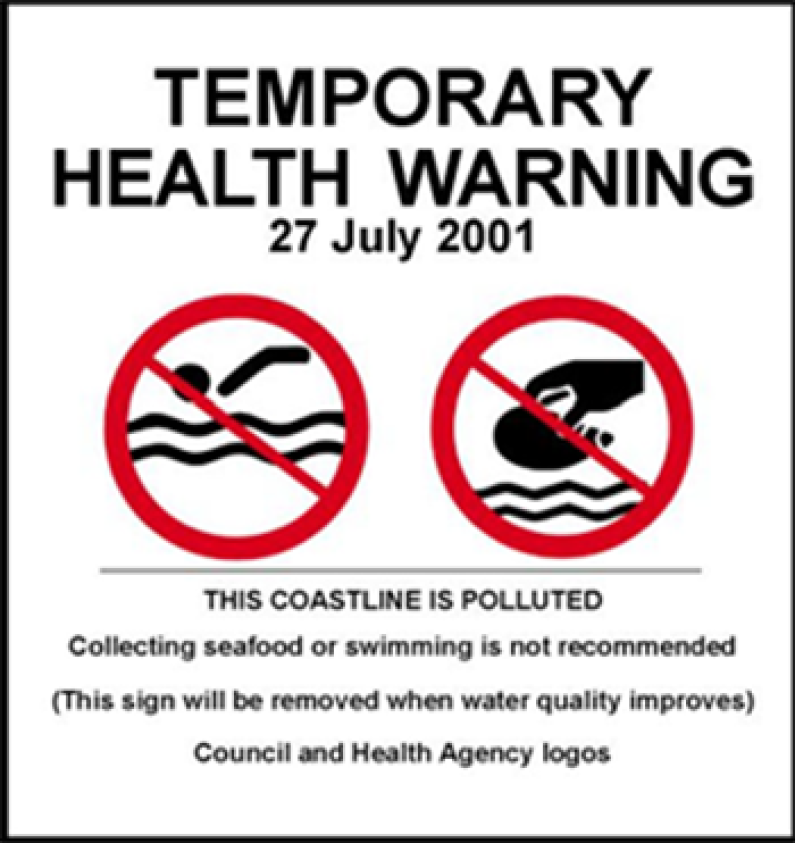
Permanent health warning sign
These signs should be erected at sites where faecal material is known to be contaminating the water on an ongoing basis. Generally these sites will either not be graded, or will be graded as Very Poor and are therefore unlikely to be monitored regularly until improvements are made.
Dimensions for these signs may vary, but they should be clearly visible at all times.
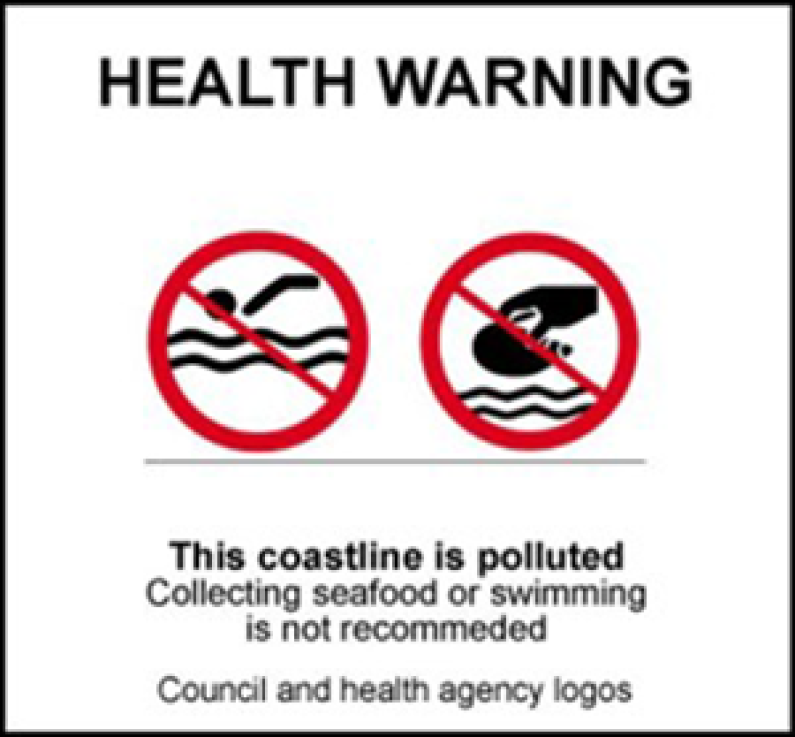
Jacobs Estuary – Southland
Environment Southland co-ordinates recreational water-quality monitoring in the Southland region. The following case study focuses on one of 13 marine recreational sites monitored in the region, details the process for developing a grade for the site, and defines the resulting surveillance monitoring regime and responses.
Microbiological Assessment Category (MAC)
Jacobs Estuary has been monitored for at least five years, so there is sufficient data to generate a MAC. Using the Hazen method recommended in the guidelines, the 95th percentile for this site is 99. Repeat or follow-up samples are not included when calculating the MAC.
Referring to the Microbiological Assessment Category Definitions the MAC for Jacobs Estuary is B.
Sanitary Inspection Category
The following table presents the findings after working through the Catchment Assessment Checklist and the resulting Sanitary Inspection Category.
Table 11: Example of completed Catchment Assessment Checklist [PDF, 11 KB]
The primary source of potential contaminants to the bathing site in Jacobs Estuary is the Aparima River. The land adjoining the Aparima River has moderately intensive agriculture with an increasing amount of dairy farming in the catchment. This corresponds to a ‘moderate’ risk. Stormwater drains enter Jacobs Estuary, but these are distant from the bathing sites and were not considered to be significant influences. A sewage overflow was discovered on the western side of Jacobs Estuary in 2001, but this did not appear to influence monitoring results and has since been rectified.
The Regional Council confirmed its catchment assessment of the site with the relevant territorial local authority and the Medical Officer of Health. The resulting Sanitary Inspection Category (moderate) was referenced from the Sanitary Inspection Flowchart.
Suitability for Recreation Grade
The suitability for recreation of this marine bathing water site was determined by integrating the site’s Sanitary Inspection Category with the site’s Microbial Assessment Category according to the Suitability for Recreation Grade matrix. The resulting grade for this site is Good.
The factors that contributed to the SFRG for this site can be summarised as follows.
Site ID | 13 |
---|---|
Site name | Jacobs Estuary |
Map reference | D46:260 168 |
SIC | Moderate |
MAC | B (95 percentile = 99) |
SFRG | Good |
Monitoring frequency | Weekly |
Boyes Beach, Bay of Plenty
Environment Bay of Plenty monitors marine and freshwater recreational areas throughout the Bay of Plenty region. This case study examines a freshwater site graded using these guidelines, including how each component is assessed and brought together to achieve an overall grade for the site.
Microbiological Assessment Category (MAC)
Boyes Beach has been monitored for two years, giving insufficient data to generate a final grade. However, there is sufficient data to generate an interim grade. An interim grade is used primarily for management purposes and must be reviewed annually as new data becomes available, until five years’ data has been collected.
The 95th percentile for this site, generated using the Hazen Method is 207. Therefore the resulting Microbiological Assessment Category is B.
Sanitary Inspection Category
The results of the catchment assessment are summarised in the following table.
Table 12: Example of a completed Catchment Assessment Checklist [PDF, 13 KB]
The primary source of potential contamination for Boyes Beach is septic tanks. In addition to the residential on-site disposal systems there is a toilet block to the rear of the beach, which is another source of faecal contamination, particularly during the holiday season when the area experiences seasonal population growth.
The land surrounding Boyes Beach is a mixture of low-density urban and low-intensity agriculture, including beef, deer and sheep. Stock have unrestricted access to waterways that discharge onto the beach, although these are not considered significant impacts due to low stock densities. There is also some forest area around the lake with the potential for run-off from feral animals. However, this was also not considered a significant source of faecal contamination.
Using the Sanitary Inspection Flow Chart for Freshwater Sites, the Sanitary Inspection Category (SIC) for Boyes Beach is Very High.
Suitability for Recreation Grade
The Suitability for Recreation Grade (SFRG) for this site is determined by integrating the site’s Sanitary Inspection Category and Microbiological Assessment Category according to the Suitability for Recreation Grade Matrix for Freshwater. The resulting grade for this site is Follow Up. This is due to the disagreement between the MAC and the SIC.
Investigation of both the MAC and SIC is recommended. The grade for this site is also interim as there are fewer than 100 data points and less than five years’ data. This is likely to be the cause of the disagreement between the MAC and the SIC also.
Factors that contributed to the SFRG for this site can be summarised as follows.
Site name | Boyes Beach |
---|---|
Map reference | U16:0380-3240 |
SIC | Very High |
MAC | B (95 percentile = 207) |
SFRG | Follow Up (Interim) |
Monitoring frequency | Continue to monitor weekly during peak usage until five years’ data has been collected and an SFRG can be assigned. |
In this appendix we have provided a series of fact sheets about recreational water-quality monitoring. Please feel free to photocopy and distribute these to anyone wanting more information about this issue.
Beaches and rivers are important
Every weekend thousands of people flock to beaches, lakes and rivers around the country and take the plunge. The numbers soar during the summer holidays. Whether it is swimming, diving, surfing or sailing, when the sun shines Kiwis head for the beach.
A part of New Zealand culture
The Hillary Commission’s 1991 Life in New Zealand Survey looked at recreational water use and found that in the 15–18-year age groups:
- 28% had been swimming in the past four weeks
- 31% had been to the beach in the past four weeks
- 23% of males and 8% of females had been either surfing or wind surfing in the past four weeks
- 20% had been involved in water-based leisure or activities in the past four weeks.
This represents only a small slice of New Zealand’s population but is a strong indicator of how our beaches are a significant part of Kiwi culture.
Mostly clean, but ...
New Zealand’s ‘clean green image’ extends to our oceans, lakes and rivers. The perception many of us have that our coasts and freshwaters are some of the cleanest in the world is well founded. We don’t have the severity of problems facing nations such as the United Kingdom or the US. But we do have some problems.
Some of our stormwater and sewerage systems are approaching 100 years old. Combined sewerage and stormwater pipes can sometimes pump untreated human effluent into lakes, rivers and the sea, especially in urban areas. Stormwater (urban run-off) collects waste from streets, lawns and parks and deposits it in waterways. This run-off often contains animal waste. Run-off from farmland (rural run-off) collects waste from farm animals, which also ends up in rivers and finally in the sea or lakes. Animal and human waste contains disease-causing organisms that may survive in the marine environment, posing a risk to human health.
What is being done about it?
Councils monitor water quality according to guidelines developed by the Health and Environment Ministries. The purpose of monitoring is to identify risks to public health from disease-causing organisms, and to inform the public of these risks. This information allows people to make informed decisions about where, when and how they use the aquatic environment for recreational activities.
Aside from monitoring water quality, many councils have long-term plans in place to deal with ageing sewerage and stormwater systems and carry out routine inspections of these systems. Regular inspection ensures the systems are maintained to as high a standard as possible so that the waters receiving the discharge meet acceptable health criteria. But it is important to realise there has always been a health risk while in contact with waters that receive stormwater, rural run-off and sewage.
Health impacts of contaminated recreational water
There are a number of disease-causing bugs (called pathogens) that once discharged into the marine and freshwater environments can survive for some time. Every time we come into contact with waters that have been contaminated with human and animal faeces, we expose ourselves to these bugs and risk getting sick. Pristine waters are unlikely to present a health risk from these pathogens.
What does ‘risk’ mean?
The risk is of getting sick when swimming, surfing or otherwise being exposed to freshwater or seawater. The guidelines that New Zealand councils use are based on fixed levels of risk, which in turn are based on overseas guidelines (which have been confirmed by New Zealand studies). Overseas investigations have settled on a maximum acceptable level of risk for marine waters of 19 in every 1000 bathers contracting an illness. For freshwaters the accepted level of risk is 8 in every 1000 bathers contracting an illness.
Even when beaches and rivers meet the guidelines there will still be a health risk associated with recreational activities in the sea. Because scientists are not directly measuring the pathogens, it is not possible to say there is zero risk to public health, especially where there are known inputs of human and animal faeces.
If tested waters exceed the acceptable level of risk, the public is advised that the area is unsuitable for recreational activities.
What does ‘illness’ refer to?
Illnesses related to contact with recreational waters were initially thought to be confined to gastrointestinal illness such as salmonellosis. More recently Giardia, Campylobacter and Cryptosporidium have also been shown to cause gastrointestinal illness. These pathogens cause diarrhoea and sometimes vomiting associated with ‘tummy bug’ symptoms. Recent studies indicate that respiratory illnesses, such as those that cause cold and flu-like symptoms, can also result from swimming in sewage-contaminated water. Skin, eye and ear infections can also be caught through contact with marine and fresh waters.
Illnesses related to toxic substances – such as heavy metals or PCBs – are not measurable with indicator bacteria and are not covered in this fact sheet.
Risk factors
Stormwater, and treated and untreated sewage discharged directly into our oceans, rivers and lakes, can contain a variety of micro-organisms that can cause disease. Therefore there may be a greater risk of getting sick under certain conditions, such as:
- two to three days after rainfall
- during full immersion in water that may be contaminated with sewage or run-off
- high exposure to shallow water where there may be higher concentrations of pathogens.
There may also be a greater risk to those with reduced immunity, the elderly and the very young.
When do the guidelines not apply?
In most communities the effluent produced will contain pathogens all year round, and the guidelines councils use are designed for use under such conditions. However, when there is an outbreak of a potentially waterborne disease in the community, and where that community’s sewage is discharged directly into or close to recreational waters without adequate treatment, the guidelines that councils use are not suitable. Under these circumstances an area may be deemed suitable for recreational use based on the indicator bacteria, when in fact the levels of pathogens are elevated to a point where there is an unacceptable risk to public health.
What are councils doing?
Councils around the country monitor water quality to minimise the risk to public health. They do this by measuring the number of enterococci (indicator bacteria) in our water. They do not measure pathogens directly because the science to do this cost effectively and reliably isn’t yet available.
In addition to measuring indicator bacteria, councils look at the area surrounding the beach or river and identify potential sources of contamination. This helps councils assess the potential risk of contamination, so they can ‘grade’ recreational sites for their suitability for recreational use.
Where do I get more information?
- Councils monitor water quality and will have detailed information on specific beaches. There may be an 0800 number to call for this information.
- Local papers and radio stations may also provide information in their community bulletins.
- Signs at monitored beaches will have details about where to get more information (0800 number, radio stations, etc.) and will indicate if the beach is suitable for recreational use.
- Some council websites have information about monitoring programmes locally.
The water quality monitoring programme
Regional, city and district councils have been monitoring water quality at beaches for many years. The guidelines now used by the councils are the Microbiological Water Quality Guidelines for Marine and Freshwater Recreational Areas published by the Ministry for the Environment and the Ministry of Health in 2002. These guidelines aim to establish national consistency in recreational water quality and public awareness of when there is a health risk.
Which beaches are being monitored?
The councils decide which freshwater and marine sites they will monitor before the bathing season begins. How councils decide this varies from region to region. Contact your local city or regional council to find out which sites in your region are monitored.
When are beaches monitored?
Beaches and rivers are monitored by the council on a weekly basis throughout the bathing season. The bathing season is generally from 1 November to 31 March, although this may vary depending on the local climate.
What do councils ‘do’ when they monitor water quality?
There are two parts to the council’s monitoring programme. Firstly, an overall grade for the site is worked out based on previous years’ monitoring results and an assessment of potential sources of contamination from the surrounding area. This grade gives an idea of the general condition of the site on any given day.
In addition to the overall grade, councils monitor recreational waters on a weekly basis during the bathing season. Samples are collected from monitored sites and tested for bacteria that indicate whether disease-causing micro-organisms, or pathogens, are present. The councils use the ‘traffic light’ approach of the guidelines to decide whether the beaches and rivers are suitable for swimming.
Surveillance monitoring is carried out when levels of indicator bacteria are low. Sites in surveillance mode are monitored weekly. The purpose of surveillance monitoring is to routinely check that water quality is at a level that doesn’t pose an unacceptable risk to public health.
The alert or ‘amber’ level acts as a trigger for councils to collect samples more frequently. The guidelines also recommend that when in this mode councils investigate whether there is a source of contamination causing the higher indicator levels at the beach or river.
For marine waters, when a sample taken during either surveillance monitoring or while in alert mode exceeds the action or ‘red’ mode indicator level, a second sample is taken immediately. If this also exceeds the action-level threshold the public are informed that the beach is not suitable for swimming.
For freshwater, if a sample taken during either surveillance monitoring or while in alert mode exceeds the action or ‘red’ mode indicator level, a second sample is not required to confirm the result. This is due to the potential for greater pathogen levels and their survival in freshwater. Illnesses may range from a skin infection, to a cold, to gastrointestinal disease. In action mode, sampling may increase to daily if the cause is unknown. The guidelines specify that a catchment assessment should be carried out in the surrounding catchment. This involves the council checking the sewerage and stormwater systems for leaks, illegal connections and other causes of contamination of the site. A catchment assessment can also involve investigating further up the catchment to identify contaminating activities or discharges.
How do I know if it is OK to swim?
Councils have communication strategies as part of their monitoring programmes to ensure the public are informed of a health risk at a beach or river as soon as possible. If you are concerned about water quality at beaches or rivers in your area, contact your local council for more information.
The bugs
In New Zealand we are becoming increasingly aware of the potential health risks associated with swimming (and other related activities) in marine and fresh waters. Although this problem is not as severe as in many other countries, it is important that we manage the problem in the interests of public health.
When monitoring water quality at New Zealand beaches and rivers, water managers and scientists are interested in the number of disease-causing pathogens present. It is impractical to measure the pathogens directly, so indicator bacteria are used to alert water managers to possible health risks presented by the pathogens.
What are indicator bacteria?
In the case of the recreational water-quality guidelines, the indicator bacteria are enterococci for marine waters and E. coli for freshwaters. These bacteria occur naturally in the gut of humans and animals, including mammals, birds, fish and reptiles. The indicator bacteria themselves do not pose a significant risk to human health. Rather, they indicate the presence of faecal material, which contains disease-causing pathogens. It is the number of enterococci or E. coli per 100 mL of water that is measured and on which the guideline levels are based.
What are pathogens?
Pathogens are microscopic organisms that cause disease in humans and animals. There are many different kinds. Some of the more widely known are Campylobacter, Salmonella, Giardia, Cryptosporidium, and viruses that cause diarrhoea and cold and flu-like symptoms. These pathogens are present in faeces and may enter our waterways through untreated sewage discharges, and from leaky sewerage pipes, septic tanks, stormwater and rural run-off.
When do the guidelines not apply?
In most communities the effluent produced will contain pathogens all year round, and the guidelines councils use are designed for use under such conditions. However, when there is an outbreak of a potentially waterborne disease in the community, and where that community’s sewage is discharged directly into or close to recreational waters without adequate treatment, the guidelines councils use are not suitable. If the guidelines are used under these circumstances a beach or river may be deemed suitable for recreational use based on the indicator bacteria, when in fact the levels of pathogens are raised to a point where there is an unacceptable risk to public health.
Indicator bacteria tell us about the likelihood of there being pathogens present in the water. We don’t use indicator bacteria to measure the likelihood of there being toxic contaminants or harmful poisons such as heavy metals, poly-aromatic carbons or PCBs.
The sources of contamination in waters used for recreation
This fact sheet discusses the sources of bacteria and pathogens in waters used for recreation that pose a risk to human and animal health.
Sewage
Sewage from many communities is collected via a network of pipes and pumped to a central facility, where it is treated before being discharged to the environment. Sewage consists mostly of water, but also contains organic material and micro-organisms. There are a number of factors that can cause sewage to enter the environment before completing its journey through the treatment facility. The main ones include:
- broken or leaky pipes – these can be caused by ageing pipes, construction activities or road works
- overflows, during heavy rainfall – the treatment facility may not be able to cope with the volumes of water and sewage entering the system, so pumps are turned off and sewage is discharged directly to the environment. This is made worse where houses have stormwater illegally connected to wastewater. Also, some older sewerage systems do not have completely separated sewage and stormwater pipes. During high rainfall, stormwater (urban run-off) can enter the sewerage system and cause sewage to overflow into the stormwater pipes. Sewage is then discharged directly to the environment
- emergency overflows – these can also occur periodically during maintenance of sewerage systems.
There are also other sources of sewage.
- Some older properties, especially baches, are still connected to a septic tank. Where these have deteriorated they leak contaminated water into the groundwater system. This contaminated water then ends up in waterways and eventually at the beach.
- Some boaties discharge their waste directly into the sea, without treatment.
The cost of upgrading much of the country’s ageing pipes to cope with our increasing population is estimated to be several billion dollars. It is anticipated that these upgrades will be spread over several decades.
Stormwater
Rainwater collected from roofs, driveways, roads, carparks and other sealed surfaces is piped directly to rivers, lakes and the marine environment without treatment. During its travels stormwater collects a number of nasties along the way. This includes waste from domestic animals, which collects on footpaths, gutters and lawns. This contaminated water is washed into the stormwater system during rainfall and ultimately ends up on our beaches. Animal waste collected in stormwater contains disease-causing organisms that can affect human health. These pathogens can cause colds, flu, respiratory illness, gastrointestinal illness and skin, eye and ear infections.
Rural run-off
Farm run-off can add significantly to the contamination of waterways. Apart from farm oxidation ponds, which have been shown to contain high levels of bacteria and pathogens, a large amount of animal waste ends up on the paddock. Depending on a number of factors – including distance to the nearest stream, rainfall intensity and stock numbers – faecal material ends up in rivers and streams, which ultimately discharge to the sea. Where stock have access to streams and rivers for drinking water, excrement may be deposited directly into the water.
Appendices
June 2003
© Ministry for the Environment